Testate Amoeba Species- and Trait-Based Transfer Functions for Reconstruction of Hydrological Regime in Tropical Peatland of Central Sumatra, Indonesia
- 1J.F. Blumenbach Institute of Zoology and Anthropology, University of Göttingen, Göttingen, Germany
- 2Department of General Ecology and Hydrobiology, Lomonosov Moscow State University, Moscow, Russia
- 3A.N. Severtsov Institute of Ecology and Evolution RAS, Moscow, Russia
- 4Department of Microbiology, Epidemiology and Infectious Diseases, Penza State University, Penza, Russia
- 5Department of Palynology and Climate Dynamics, University of Göttingen, Göttingen, Germany
- 6Department of Soil Science, University of Jambi, Jambi, Indonesia
- 7Department of Soil Science and Land Resource, Bogor Agricultural University (IPB), Bogor, Indonesia
Tropical peatlands play an important role in carbon storage and in water regulation on a landscape level. However, our understanding of their ecology and long-term hydrological dynamics remains limited. Transfer functions, constructed on the basis of biological indicators (proxies) with known ecological preferences, allow us to infer past environmental conditions and serve as a basis for prediction of future changes in peatlands. Here, we use testate amoebae to develop the first species- and functional trait-based transfer functions for the Southeast Asia. This provides a valuable tool for future reconstructions of past hydrological changes in tropical peatlands, their development, and climatic changes. Surface samples for testate amoeba analysis were taken in various biotopes along two transects across the Sungai Buluh peatland in Central Sumatra. The following environmental characteristics were measured: water table depth (WTD), light intensity, pH, total C and N concentrations. The analysis of the surface samples revealed 145 morphotypes of testate amoebae belonging to 25 genera. A significant fraction of the variance in testate amoeba morphotypes and functional trait composition was explained by WTD and pH. The wide WTD range (0–120 cm) seems more valuable for reconstruction than the extremely short pH gradient (2.5–3.8). Thus, transfer functions were developed only for WTD, based on weighted averaging model for morphotypes and multiple linear regression for functional traits. Both species- and trait-based model have a predictive ability for WTD reconstruction. For traits, the best performance of the model was reached by including five morphological traits: shell width, aperture shape, aperture invagination, shell shape and shell compression. We discuss the ecology of several taxa and highlight the traits, which reflect hydrological changes in this system. Though the hydrological preferences of some species are similar to those in high and middle latitude peatlands, we argue that latitudinal differences in morphospecies composition and variations in environmental relationships of species require the development of region-specific transfer functions. Moreover, our results indicate that ecological preferences of morphotypes within morphospecies also need to be considered and included in future studies.
Introduction
Tropical peatlands play a crucial role in carbon sequestration, regulating water resources and safeguarding rich biodiversity (Page et al., 2011; Posa et al., 2011; Hapsari et al., 2017). These peatlands, however, are vulnerable to climate changes and threatened by their extensive conversion into agricultural production systems (Page et al., 2011; Miettinen et al., 2012, 2013). About a century ago, Polak (1933) wrote that the study on peatlands in the tropics was still at an early stage. This statement, unfortunately, is still true to this day for the Asian tropics, in particular for palaeoecological research in the region (Biagioni et al., 2015). Facing the current changes, there is the pressing need to study these “palaeoenvironmental archives” (Jackson and Charman, 2010) to evaluate past environmental conditions and to predict future changes of these ecosystems. However, crucial steps in palaeoenvironmental reconstructions are the development of a representative present-day organisms-environment training set and the application of adequate numerical methods to model the relationship between the occurrence and abundance of recent organisms and their environment (Juggins and Birks, 2012). Using these so-called transfer functions allows a quantitative estimation of past environmental conditions. Transfer functions have been frequently developed and applied to predict temperature, precipitation, sea level, pH and water table depth based on species composition of pollen, chironomids, diatoms, and testate amoebae in lake and mire sediments across many regions (Birks et al., 1990; Gehrels, 2000; Wilmshurst et al., 2003; Charman and Blundell, 2007; Klemm et al., 2013; Massaferro and Larocque-Tobler, 2013).
Testate amoebae are common organisms in many mires and, due to their test (shell), they are often preserved in peats (Swindles and Roe, 2007; Mitchell et al., 2008a). Diverse shell morphological characteristics and prominent environmental preferences make testate amoebae valuable ecological indicators. Previous studies showed that species composition of testate amoeba communities are controlled by surface wetness (often estimated as water table depth), pH, temperature and sea-level change (Charman, 2001; Mitchell et al., 2008a).
A number of transfer functions have been developed based on the relationship between testate amoebae and peatland hydrology in the Northern Hemisphere. For summarised studies on transfer functions from North America and Europe see, for example, Amesbury et al. (2016, 2018). However, only few transfer functions were developed for the Southern Hemisphere (Wilmshurst et al., 2003; Swindles et al., 2014, 2018a; van Bellen et al., 2014). In part, this might be due to the fact that information on ecological preferences of testate amoebae in tropical peatlands is extremely limited. However, data obtained from peatlands of temperate and polar regions may not be applicable to low-latitude peatlands (Charman, 1997).
Traditionally, the construction of transfer functions is species-based, which is difficult if identification of the taxa is hampered (Mitchell et al., 2014). Also, the environmental preferences of many species are not well known and this restricts their use as ecological indicators (Charman, 1997). Alternatively, ecologically significant morphological and physiological functional traits may serve as indicators of the environmental conditions the organisms live in Fournier et al. (2015), van Bellen et al. (2017), and Koenig et al. (2018). Thus, functional traits may provide powerful links to ecosystem processes and help to strengthen the transfer functions approach based on taxonomic information only (Fournier et al., 2015; Lamentowicz et al., 2015; van Bellen et al., 2017). In recent years, a new approach for reconstructing past environmental conditions based on testate amoeba functional traits has been developed and successfully applied (for details, see Marcisz et al., 2020, submitted). However, the approach still needs refinement and this applies in particular to tropical peatlands. Therefore, this study aims at (1) extending knowledge on testate amoeba communities in Sumatra, (2) evaluating the relationships among testate amoeba morphospecies, functional traits and environmental variables, and (3) developing species- and trait-based transfer functions for future palaeoecological reconstructions of hydrological regime.
Materials and Methods
Study Site
The Sungai Buluh peatland is a protected forested swamp which covers an area of 18,000 ha. It is located approximately 19 km from the coastline and 30 km north-east of the city of Jambi in Central Sumatra with an elevation ranging from 9 to 25 m above sea level (Hapsari et al., 2017). From the geomorphological and hydrological point of view, the peatland of Sungai Buluh is an extensive coastal peat dome, which is delimited by two rivers located to the east and west sides. The mouths of the rivers are located on the coast about 20 km north to the peatland and are influenced by strong diurnal tides. Peat depths at the study site (measured with the Peat Probe to the mineral layer) vary from 216 to 720 cm (mean 430 cm).
The Sungai Buluh area is covered with a secondary peat-swamp forest, which has been regenerating after selective logging in the 1960s and 1970s (Nurjanah et al., 2013). In 1997, El Niño related fires reduced the forest canopy (Tata et al., 2016) so the seedlings of the tree species Shorea pauciflora and Dyera polyphylla were planted in 2003 (Nurjanah et al., 2013). The Sungai Buluh peatland is surrounded by agricultural fields and plantations, for example, pulp wood Acacia spp. and oil palm Elaeis guineensis (Melati et al., 2015).
The climate of the area is tropical humid. There is little temperature variation throughout the year with mean annual temperature of 26°C. The average annual precipitation in the area is 2400 mm with a slightly drier season from June to September (Aldrian and Susanto, 2003; Karger et al., 2016). The inter-annual variability of rainfall is controlled by the El Niño Southern Oscillation (Aldrian and Susanto, 2003) and the Indian Ocean Dipole (Saji et al., 1999). While the precipitation patterns of the area are influenced by the Asian-Australian monsoon and the Intertropical Convergence Zone (Saji et al., 1999).
Field Work
For the development of the training set, surface samples of testate amoeba communities were collected along two transects (NS and WE) across the peatland on August 3 – 6, 2016 (Figure 1). In total, 29 plots were laid out along the NS transect (total length ∼500 m) and 41 plots along the WE transect (total length ∼600 m). The distance between the NS and WE transects was about 12 km. The NS transect was located in the centre of the peatland and represented the conditions typical for the internal part of the dome with the deepest peat deposits, however, with a rapid change in land use, this is no longer visible on the map (Figure 1). On the other hand, the WE transect was located closer to one of the rivers and represented the peripheral part of the peatland characterised by minerogenic conditions. The most common plant families were Myristicaceae, Dipterocarpaceae, Anacardiaceae, Myrtaceae, Euphorbiaceaea. The most common species were Myristica lowiana, Macaranga sp., Shorea rugosa, Syzygium attenuatum, Dyera polyphylla, Gluta aptera (personal observations). These species widely occur in natural swamp rainforests of Southeast Asia and indicate successful restoration measures at the protected forest (Graham et al., 2017). Both locations were chosen because of their natural conditions and accessibility.
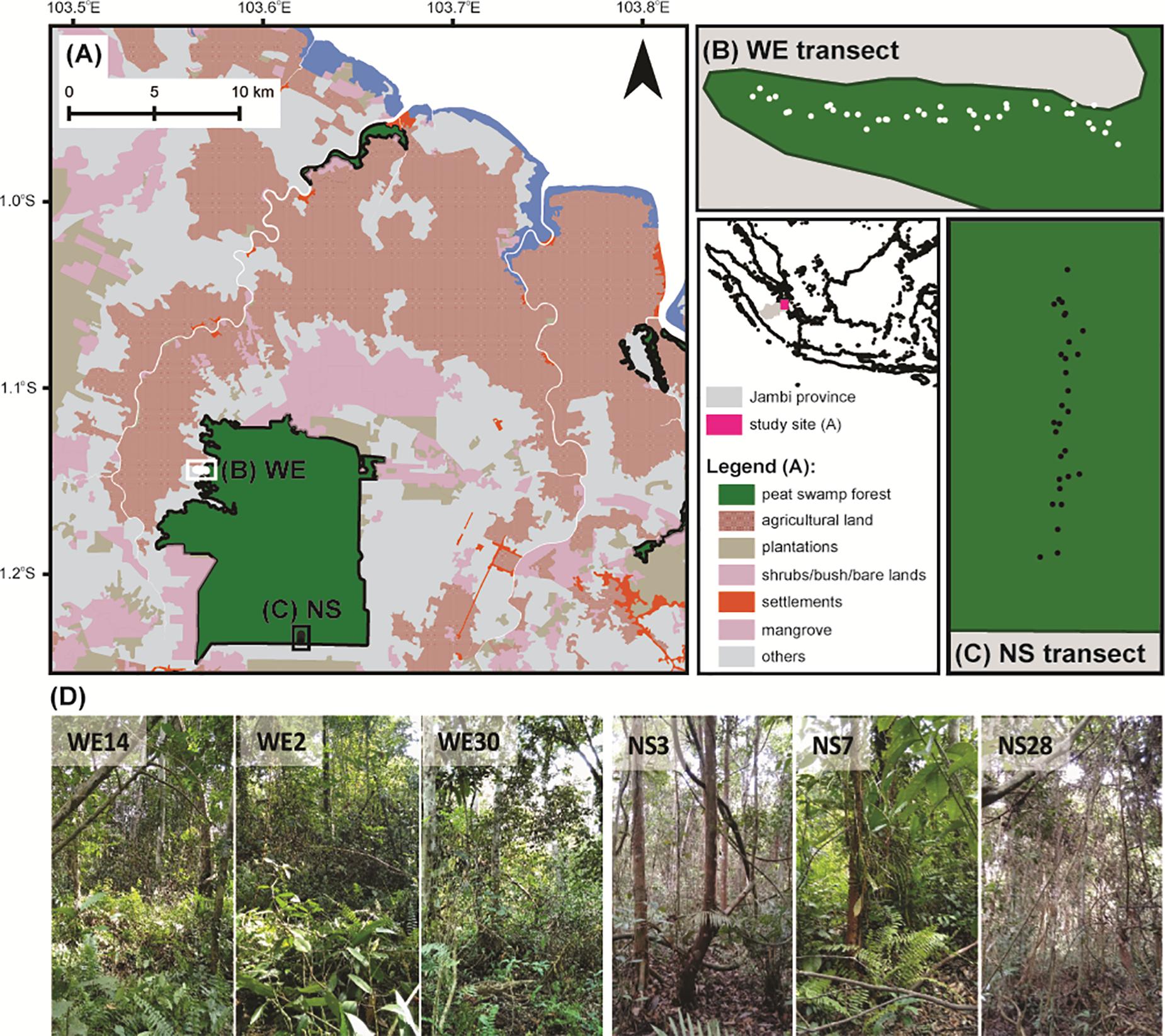
Figure 1. Map of the Sungai Buluh protected peat swamp forest (A) with studied WE (B) and NS (C) transects. Each point on the WE and NS transects indicates the sampling location. (D) Examples of some sampling locations.
The location of all 70 sampling plots was identified using the GPS of a smart phone with connectivity to GLONASS, Galileo, and Beidou location services (Aquaris X Pro model, BQ; for sampling plot details see Supplementary Table 1). At each plot, a sample of the top 50 mm litter/fermentation layer was taken with a corer (diameter 50 mm) and used for testate amoeba analysis and the determination of substrate water content, pH, and concentrations of carbon (C) and nitrogen (N). The extracted substrate of all samples was classified as tree litter, herbal litter, rooted soils or pool sediments. The samples for testate amoeba analysis were air dried (25°C) and stored at 4°C until analysis (Mazei et al., 2015). At each plot, microrelief was classified as pool, hollow, flat or hummock. The water table depths (WTD, cm) was measured in relation to the peatland surface (negative values denote submerged substrates) after augering with a corer. The measurements were repeated several times until values were stable. Light intensity (illuminance, lux) was determined using a lux meter of the camera from the same smart phone used for the GPS location (Gutierrez-Martinez et al., 2017). Despite differences in day time of measurement (from 11 am until 3 pm), light intensity is generally driven by canopy cover and the measurements, therefore, reflect light conditions at the sampling sites. The characteristics of the sampling plots are presented in Supplementary Table 1.
Laboratory Analyses
Substrate pH (CaCl2) was measured using a digital pH meter. For measuring concentrations of C and N, aliquots of the substrate were dried at 65°C for 72 h, milled and analysed using an elemental analyser (Carlo Erba, Milan, Italy). Water content of the substrate was determined gravimetrically (wet weight percentage), for details see Supplementary Table 1.
Testate amoeba analysis was performed according to the procedure described by Mazei et al. (2011). To facilitate detachment of testate amoeba shells from litter, 4–6 g air-dried material was placed in sterile tap water at 5°C for 24 h. Then, the suspension was stirred for 10 min, filtered through 500 μm mesh and left to settle at 5°C for 24 h. The settled material was analysed at 200–400× magnification using a light microscope (Zeiss Axiostar plus, Germany). A minimum of 150 shells was counted for each sample. Two samples were omitted from further analyses because of low shell numbers, i.e., NS18 (0 shells) and WE30 (17 shells).
Measurements of the Shell and Functional Traits Assignments
Most individuals could be assigned to species (morphospecies), others were listed as morphotypes, based on differences in shell size (Hoogenraad and de Groot, 1940, 1942; Bartos, 1963; Mazei and Tsyganov, 2006); for the taxa list see Supplementary Table 2. Shell size measurements were done for each specimen to assign it to a particular size class. If the size range (shell length and shell width) corresponded to the original description (see Authorship in the Supplementary Table 2), we assigned this individual to morphospecies level; if the shell length or shell width was variable, we assigned each individual into morphotypes of known morphospecies with a precise indication of the shell size for each of them and named them as “morph,” as shown in Supplementary Table 2. The total number of each measured individuals of morphotype/morphospecies is shown in Supplementary Table 2, see column named “Individuals, found in all samples”.
The selection of functional traits of testate amoebae was based on their ecological importance as indicated by previous publications (Fournier et al., 2015; van Bellen et al., 2017; Koenig et al., 2018). The initial list of functional traits included the following characteristics: (1) shell length, shell width, aperture size and biovolume (Gilbert et al., 1998), measured for each found individual and for 150 shells for each sample, see Supplementary Table 2; and (2) shell shape, shell compression, shell composition, presence of mineral particles, aperture position, aperture shape, aperture invagination, mixotrophy and order-based phylogenetic group, taken from original descriptions (see Supplementary Table 2, column Authorship). All qualitative characteristics were categorised into two to six categories (Supplementary Table 2). Briefly, for shell shape six categories were defined: circular, oviform, pyriform, oviform/elongate, oviform/pyriform and flask-shaped. For shell compression-six categories: very compressed, compressed, sub-spherical, hemispherical, spherical/cylinder and spherical. For shell composition-six: proteinaceous, siliceous, agglutinate/mineral, agglutinate/siliceous, agglutinate/mineral/siliceous, and calcareous. For mineral matter presence-two categories: present and absent. For aperture position-three: terminal, sub-terminal and central. For aperture shape-six: oval, oval/circular, circular/irregular, circular, irregular and slit-like. For aperture invagination-three: invaginated, not-invaginated and slightly invaginated. For mixotrophy-two: no and yes. For order-four: Arcellinida, Amphitremida, Euglyphida and Gromiida. Some of the traits, e.g., shell size, shell compression, aperture position were assumed to reflect substrate wetness conditions and represent adaptations to wet or dry conditions (Fournier et al., 2015; van Bellen et al., 2017), whereas shell composition (more siliceous or more proteinaceous) may indicate pH or C/N ratio, i.e., the nutrient status of the environment (Mitchell et al., 2008b). The abovementioned functional traits were determined for each morphotype and combined in data frame for subsequent calculations (Supplementary Table 2). For details on the occurrence of each trait in the samples see Supplementary Table 2. For previously undescribed morphotypes, measurable (quantitative) characteristics were assessed, non-measurable (qualitative) were taken from the known genus level after visual validation under the microscope.
Data Analyses
All calculations and statistical analyses were performed in R (R Core Team, 2018). Based on the traits, community weighted means (CWMs) were calculated for each sample, reflecting the functional composition, expressed as the mean trait value of species present in the community weighted by their relative abundance for quantitative traits (shell length, shell width, biovolume, aperture size), or the relative abundance of all taxa with the respective trait for qualitative traits (Laliberté et al., 2014). Effects of environmental variables (substrate type, microrelief, WTD, water content, pH, C-to-N ratio, and light intensity) on the morphotype and functional traitcomposition of testate amoeba communities were analysed using redundancy analysis [RDA and CWM-RDA, see Kleyer et al. (2012)] in the vegan package (Oksanen et al., 2017). Environmental parameters affecting the community composition were selected using the forward selection procedure with the function “ordistep” (package vegan). Variation partitioning was performed for the selected environmental parameters in order to estimate the contribution of each variable to the variation in community composition. The traits with the strongest linkage to WTD were selected for building the trait-based transfer functions based on their scores in RDA. A Monte Carlo permutation test (1000 iterations) was used to test the significance of the models. The relationships between quantitative environmental variables were estimated with Spearman’s rho statistic (rank-based measure of association; function “cor.test”).
Transfer functions were constructed using the main calibration methods used in palaeoecology: weighted averaging (WA), weighted averaging with tolerance down weighting (WA-Tol), where high-tolerance species are assigned lower weights than low-tolerance species to reduce their contribution to the model, partial least squares (PLS) and multivariate regression (MR) in rioja package (Juggins, 2017). Model performance was evaluated using leave-one-out (LOO) and bootstrapping (n = 1000) cross validation techniques. The best models were selected using the coefficient of determination (R2), the root mean square error of prediction (RMSEP) and maximum bias. The estimation and visualisation of morphotype optima and tolerances for the selected environmental variables was performed with the function “caterpillar” in the package analog (Simpson, 2007).
Results
General Observations
The summary statistics of the measured environmental variables along the studied transect is presented in Table 1 and in Supplementary Figure 1. The overall range of the WTD values varied from −12 to 120 cm (mean value 61.7 cm, SD = 32.5 cm) and was characterised by a bimodal distribution with peaks at around 25 and 75 cm. The overall correlation (Spearman rho value) between WTD and surface water content was −0.60 and was greater along the NS transect (−0.76) as compared to WE transect (−0.33), which was due to the fact that the WE transect was generally drier and WTD therefore less affected surface water content. Both variables were included in further analyses to evaluate their effects on testate amoeba communities. The soils were generally very acid with the pH varying from 2.5 to 3.8. Most of the sites were located under dense canopy cover with a light intensity <5000 lux. The C-to-N ratio of the substrate varied from 19.5 to 35.5.
In total, 10,217 shells were counted and assigned to 145 morphotypes of testate amoebae belonging to 65 morphospecies and 24 genera (Supplementary Table 2). The most abundant morphospecies were Hyalosphenia subflava (morph 2 – 15.6% of total counts, morph 1 – 11.6%, morph 4 – 9.1%, morph 5 – 3.1%), Hyalosphenia minuta (9.6%), cf. Cyclopyxis eurystoma v. parvula morph 2 (9.0%), cf. C. eurystoma v. parvula morph 1 (5.9%). These morphospecies were the most common and were observed in more than 85% of the samples. Thirty-two morphospecies were present in one sample only with the maximum relative abundance per sample rarely exceeding 2.0% except for Trinema complanatum v. globulosa (maximum relative abundance per sample 13.0%).
Effects of Environmental Variables on Morphotype and Functional Trait Composition
Redundancy analysis of morphotypes composition indicated that the environmental variables (substrate type, microrelief, WTD, substrate water content, pH, C-to-N ratio and light intensity) explained 29.6% (R2) of the total variance (pseudo-F = 2.4, p < 0.001, df = 10,56). Forward selection procedure identified WTD and pH as the most important environmental variables explaining 14.0% (pseudo-F = 11.1, p < 0.001, df = 1,64) and 3.4% (pseudo-F = 2.7, p = 0.02, df = 1,64) of the total variation (Figure 2). Most of the taxa were generally associated with wet acidic biotopes. Surprisingly, the morphotypes of H. subflava showed opposite relations to WTD, i.e., morph 1 and 2 were positively related to WTD, whereas morph 3, 5, 7, and 10 were negatively related to WTD. H. subflava morph 4 showed little correlation to WTD in general. Morphotypes Cyclopyxis eurystoma vs. parvula morph 1, Trinema enchelys morph 1 preferred biotopes with the upper range of pH value.
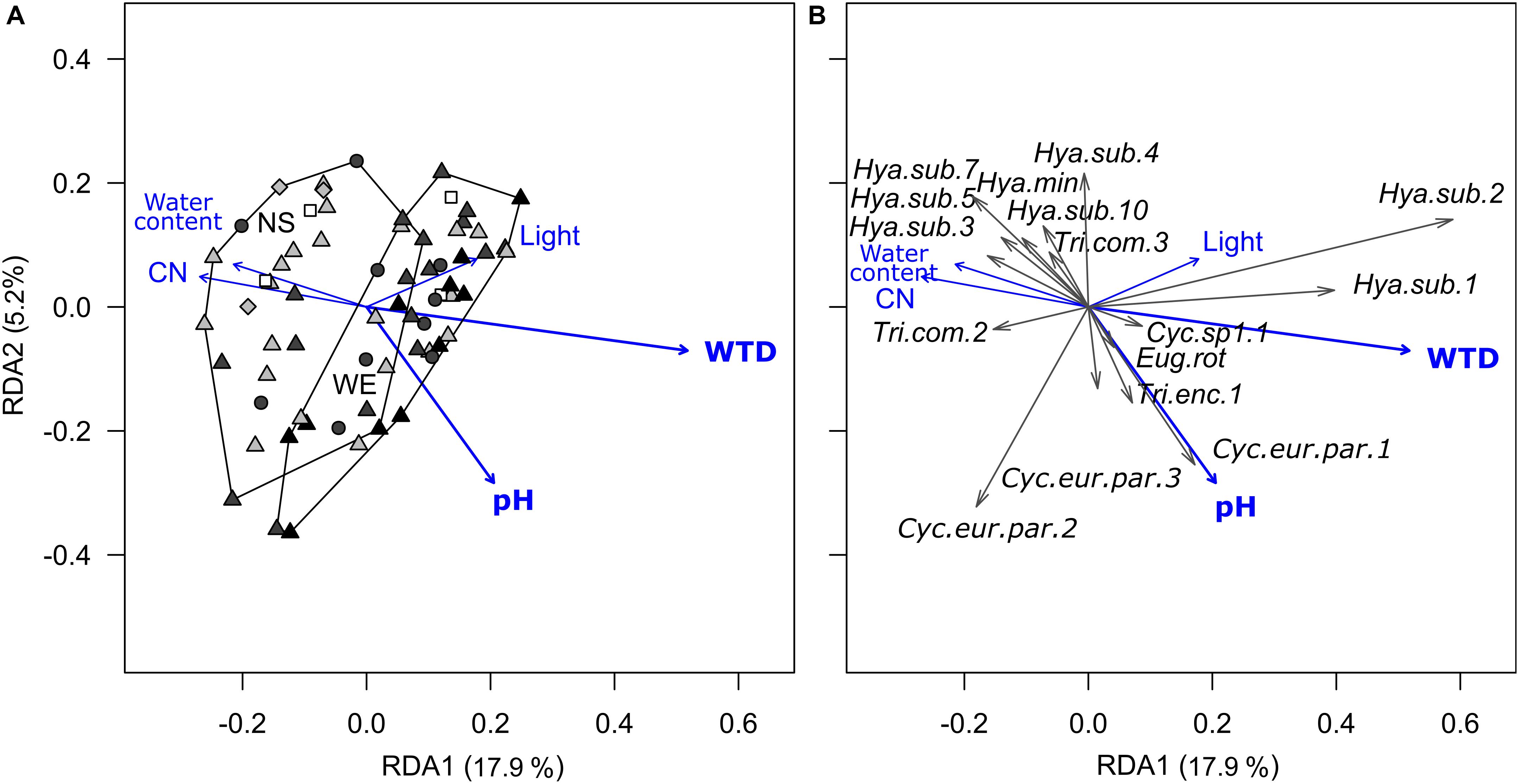
Figure 2. Ordination diagrams based on the result of RDA showing the variation in morphotype composition (unscaled data) of testate amoeba communities in surface samples from the Sungai Buluh peatland in response to environmental variables. (A) Sample scores: the polygons encircle samples from the same transect (NS or WE); symbol colour denotes the microrelief (black – hummock, dark grey – flat, light grey – hollow, white – pool), symbol shape denotes the substrate type (circle – herbal litter, square – pool sediments, diamond – rooted soil, triangles – tree litter). (B) Species scores (only values ≥ |0.04| with at least one of the axes are shown). The number in parentheses is the proportion of the total variance explained by the axis. Significant environmental variables (WTD and pH) are shown in bold. WTD, for water table depth; CN, for C-to-N ratio; Light, for light intensity. For species abbreviations, see Supplementary Table 2.
For functional traits, CWM-RDA showed similar results. The full model constrained by all environmental variables (Figure 3) explained 20% (R2) of the total variance in functional traits composition (pseudo-F = 1.41, df = 10, 56, p = 0.041). Forward selection showed that both pH and WTD should be retained for the parsimonious model, but the WTD (R2 = 2.8%, pseudo-F = 1.93, df = 1, 64, p = 0.048) explained less than pH (R2 = 5.3% pseudo-F = 3.71, df = 1, 64, p = 0.009). Based on the functional trait scores in the RDA, the strongest association with the WTD were detected for biovolume (−0.55), shell length (−0.48), oval/circular aperture (−0.45), shell width (−0.44) and sub-spherical shell compression (−0.26), i.e., in drier locations shells were smaller, sub-spherical, with oval/circular apertures (Supplementary Table 3). In addition, positive relationships with WTD were determined for oviform/elongate shells (0.35) and slightly compressed shells (0.32), i.e., they were dominating in drier locations. A number of functional traits (shell length, shell width, biovolume, oviform and elongated shell shapes and oval/circular aperture shells) were related to pH, even though it varied in a small range along the transects (for details, see Supplementary Table 3). No significant correlations were detected between any of the functional traits and light intensity, presumably because the abundance of mixotrophic species was very low (single occurrences in few samples), see Figure 3. All the above-mentioned traits related to WTD were selected for the transfer function development. The relationships between the selected functional traits and WTD were generally linear, except for shells with slightly invaginated aperture (Supplementary Figure 2). Shell width, shell length and biovolume are not independent of each other (intercorrelated traits, see Figure 3 and Supplementary Table 3), and therefore we tested several models with different combinations of these traits.
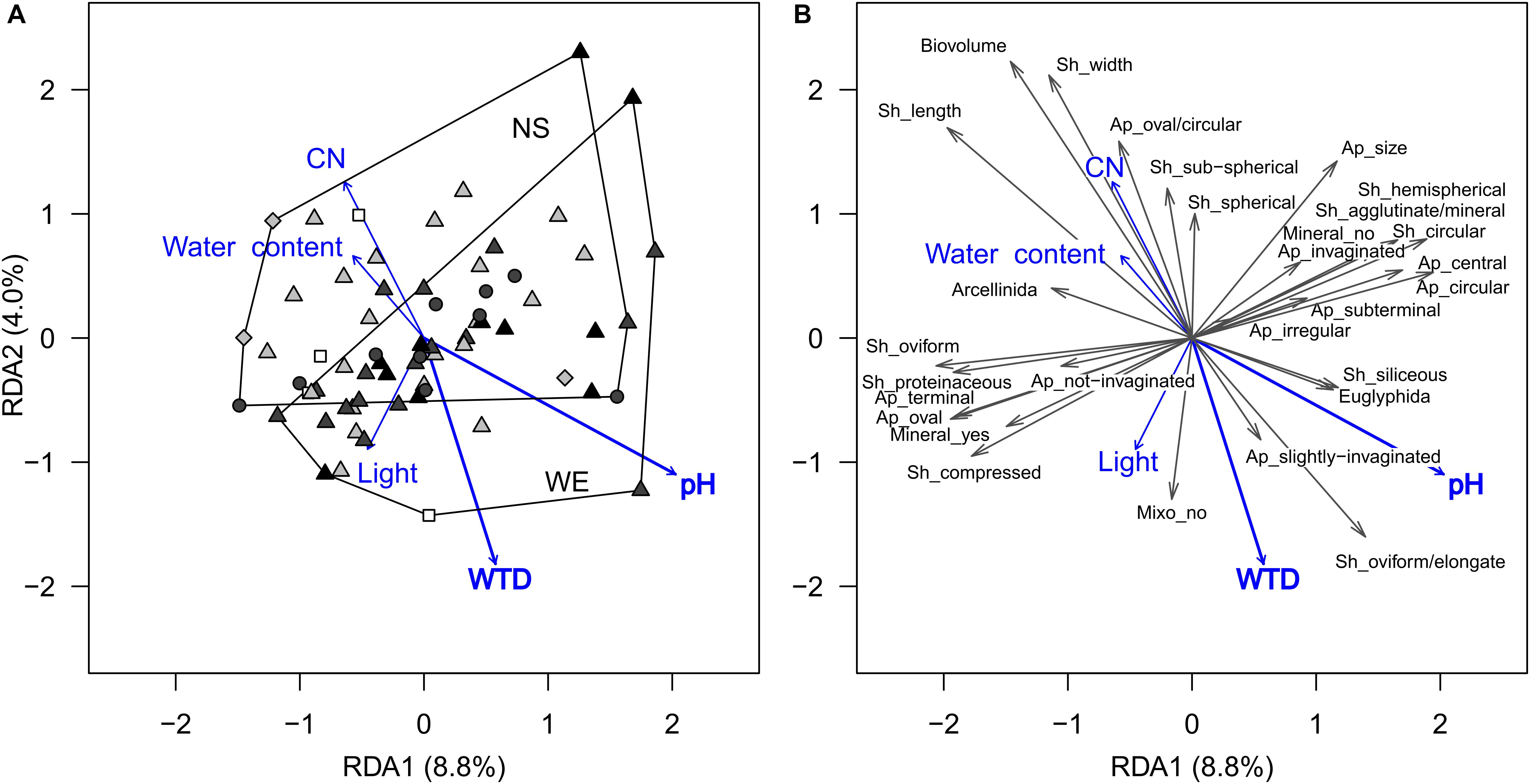
Figure 3. Ordination diagrams based on the result of RDA showing the variation in testate amoeba functional traits composition (scaled data, only traits observed in more than 15 samples are shown) in surface samples from the Sungai Buluh peatland. (A) Sample scores: the polygons encircle samples from the same transect (NS or WE); symbol colour denotes the microrelief (black – hummock, dark grey – flat, light grey – hollow, white – pool), symbol shape denotes the substrate type (circle – herbal litter, square – pool sediments, diamond – rooted soil, triangles – tree litter). (B) Functional trait scores (multiplied by 2 to improve readability). The number in parentheses is the proportion of the total variance explained by the axis. Significant environmental variables (WTD and pH) are shown in bold. Trait abbreviations: Sh – shell, Ap – aperture, Mineral – mineral presence, Mixo – mixotrophy. WTD, for water table depth; CN, for C-to-N ratio; Light, for light intensity.
Morphotype- and Trait-Based Transfer Functions
For morphotypes, the best performing transfer functions are shown in Table 2 (for the other models see Supplementary Table 4). The inverse weighted averaging model (68 samples, 143 morphotypes) showed the best performance according to the cross-validation methods (Figure 4). Depending on the cross-validation method, R2 varied between 70 and 71% and RMSEP varied between 17.7 and 18.7 cm. After screening for outliers (Figure 4), the samples with a residual greater than 25 cm (absolute value) were removed (Supplementary Table 5). This improved the performance of the transfer function (R2 = 77%, RMSEP = 15–16 cm, 63 samples, 139 morphotypes, WTD range 0–120 cm, SD = 31.4 cm). Tolerance and optimum values for the 87 morphotypes of testate amoebae were analysed and plotted in Figure 5. The functional traits were generally characterised by linear relationships to WTD and therefore were better modelled by multiple regression (MR) (Supplementary Table 6). Nonetheless, the performance of MR model for functional traits was weaker as compared to the WA inverse for morphotypes even after the removal of outliers with the residuals greater than 40 cm (absolute values; Table 2).
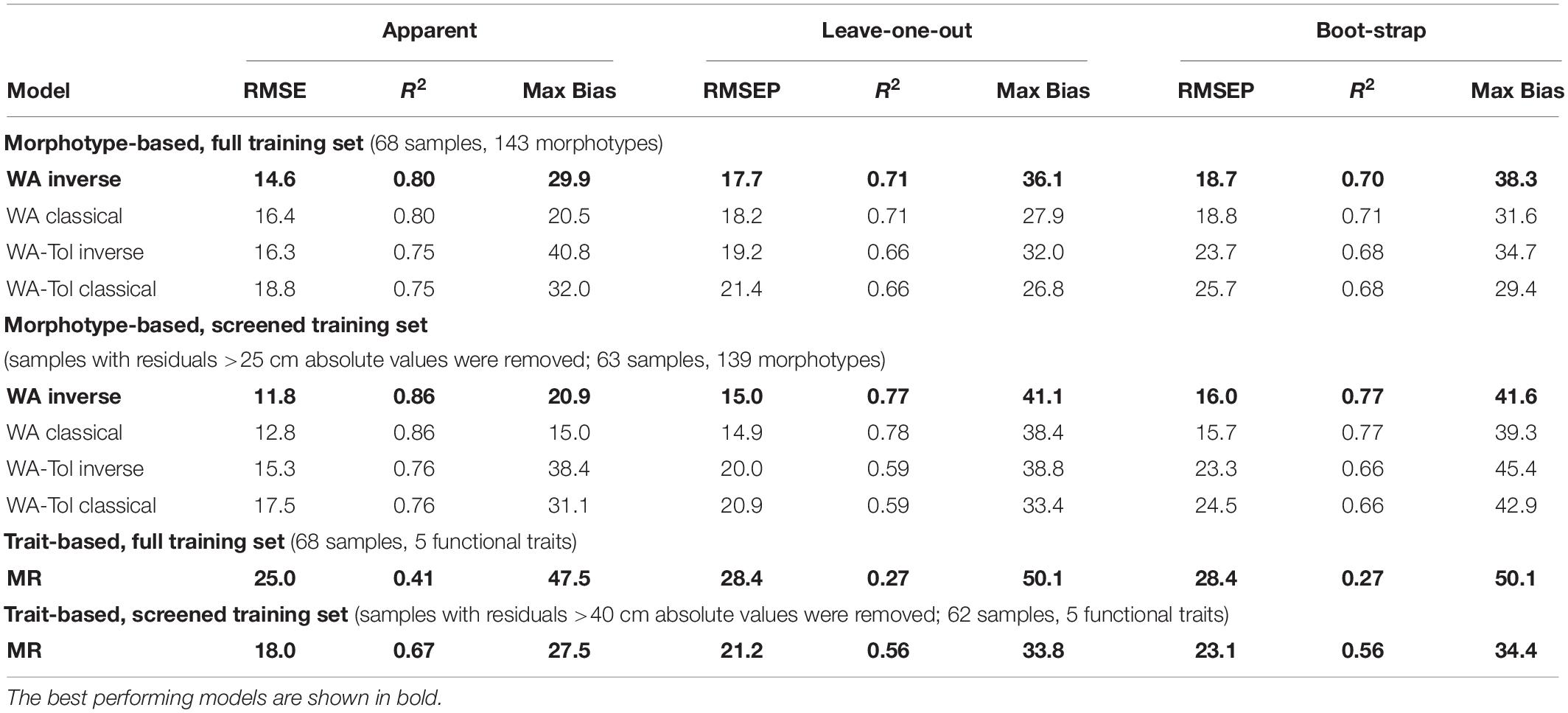
Table 2. Performance statistics for the morphotype- and trait-based transfer functions (weighted averaging (WA) and multiple regression (MR) models) for reconstruction of water table depth.
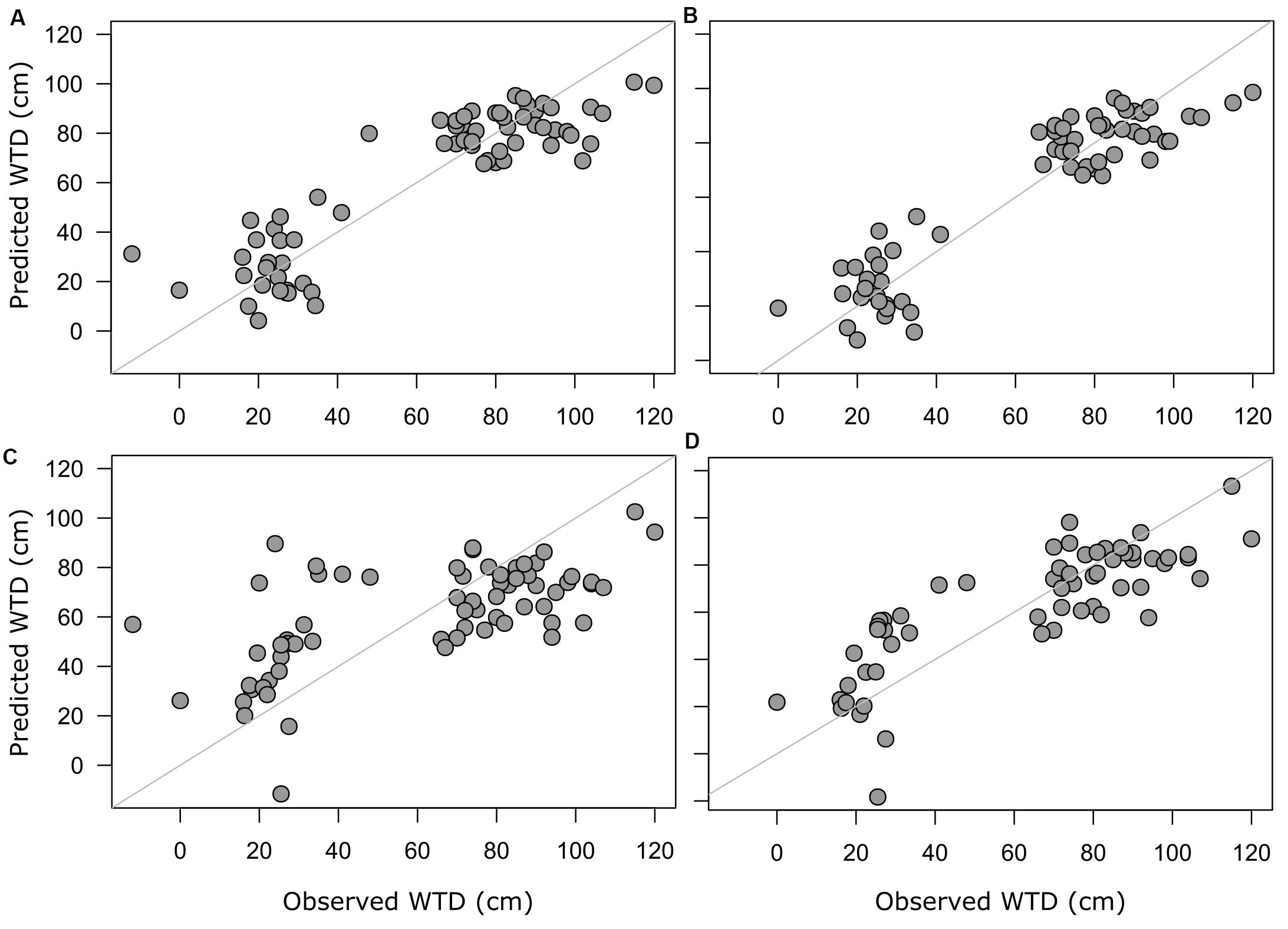
Figure 4. Predicted WTD values plotted against observed WTD values. Predicted values are based on model estimates; full training set of morphotype-based WA model (A) and screened training set with the removed outliers for morphotype-based WA model (B), the functional trait-based model (C) with selected functional traits and full training set and screened training set with the removed outliers for selected functional trait-based model (D).
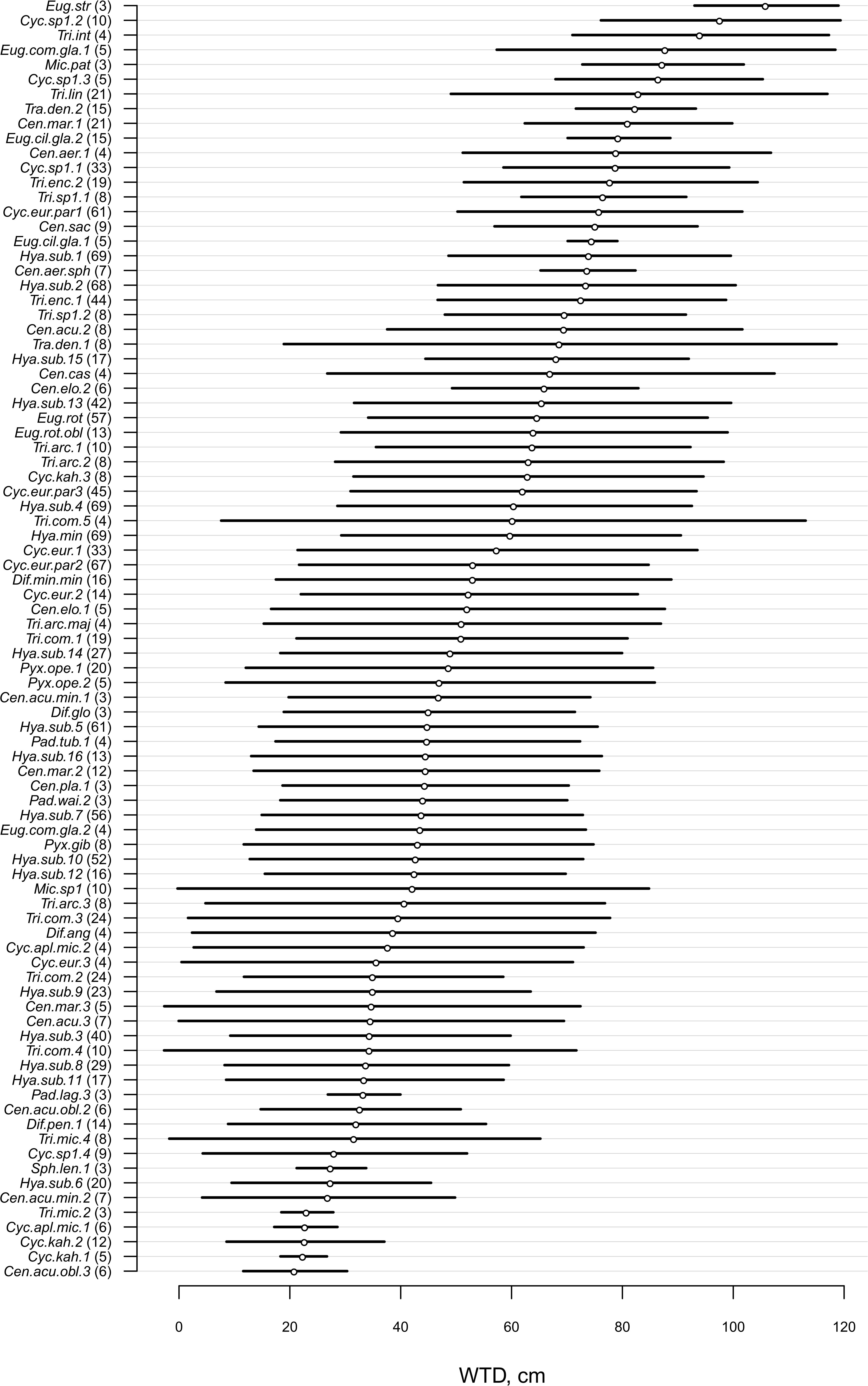
Figure 5. Water table depth (WTD, cm) optima and tolerances for 87 taxa (present in >3 samples, for the exact number, see number in brackets) based on the inverse weighted averaging model after removal of samples with high (>25 cm, absolute values) residual values. For species abbreviations, see Supplementary Table 2.
Discussion
Testate Amoeba Communities in the Sungai Buluh Peatland
In the studied peatland we found 145 taxa from 25 genera in the 70 samples taken (Figure 6). This is similar to other ecosystems in Jambi Province, including rainforests and agricultural lands, where about 150 taxa were found in litter samples (Clough et al., 2016; Krashevska et al., 2016), and similar to moss samples in Java where about 126 taxa were found (Bartos, 1963). Many of the recorded morphospecies do have a cosmopolitan distribution. However, the variability in the morphological characteristics of the shell was unusually high in a number of these morphospecies. One of the most dominant and variable morphospecies was H. subflava, suggesting that it forms a species complex (Figure 7). Shell length of H. subflava ranged from 40 to 105 μm and shell width from 32 to 75 μm. We identified 17 morphotypes in this morphospecies and these morphotypes represented about 50% of the total number of individuals of testate amoebae in our samples. This great variability, to the best of our knowledge, has not yet been documented for any other Hyalosphenia in higher latitude ecosystems, but regularly reported for other tropical regions, including Indonesia, Peru, and Panama (Hoogenraad and de Groot, 1942; Biagioni et al., 2015; Krashevska et al., 2016; Swindles et al., 2016, 2018a). H. subflava has been generally considered as an indicator of dry conditions in high- and mid-latitude peatlands (Charman et al., 2000). However, our study shows that, in tropics, this abundant and morphologically variable species may form a species complex comprising of morphotypes which differ in ecological preferences (see “Relationships of Testate Amoeba Morphospecies and Traits With Environmental Variables”). The second dominant morphospecies was a cosmopolitan species cf. C. eurystoma v. parvula (Figure 6C), which also showed variability in the shell length and potentially represented a species complex. It is noteworthy that the morphological differences between C. eurystoma v. parvula and Phryganella acropodia are difficult to detect with light microscopy. Therefore, these morphospecies should be treated with caution, especially while comparing different studies, and interpreting their indicator value.
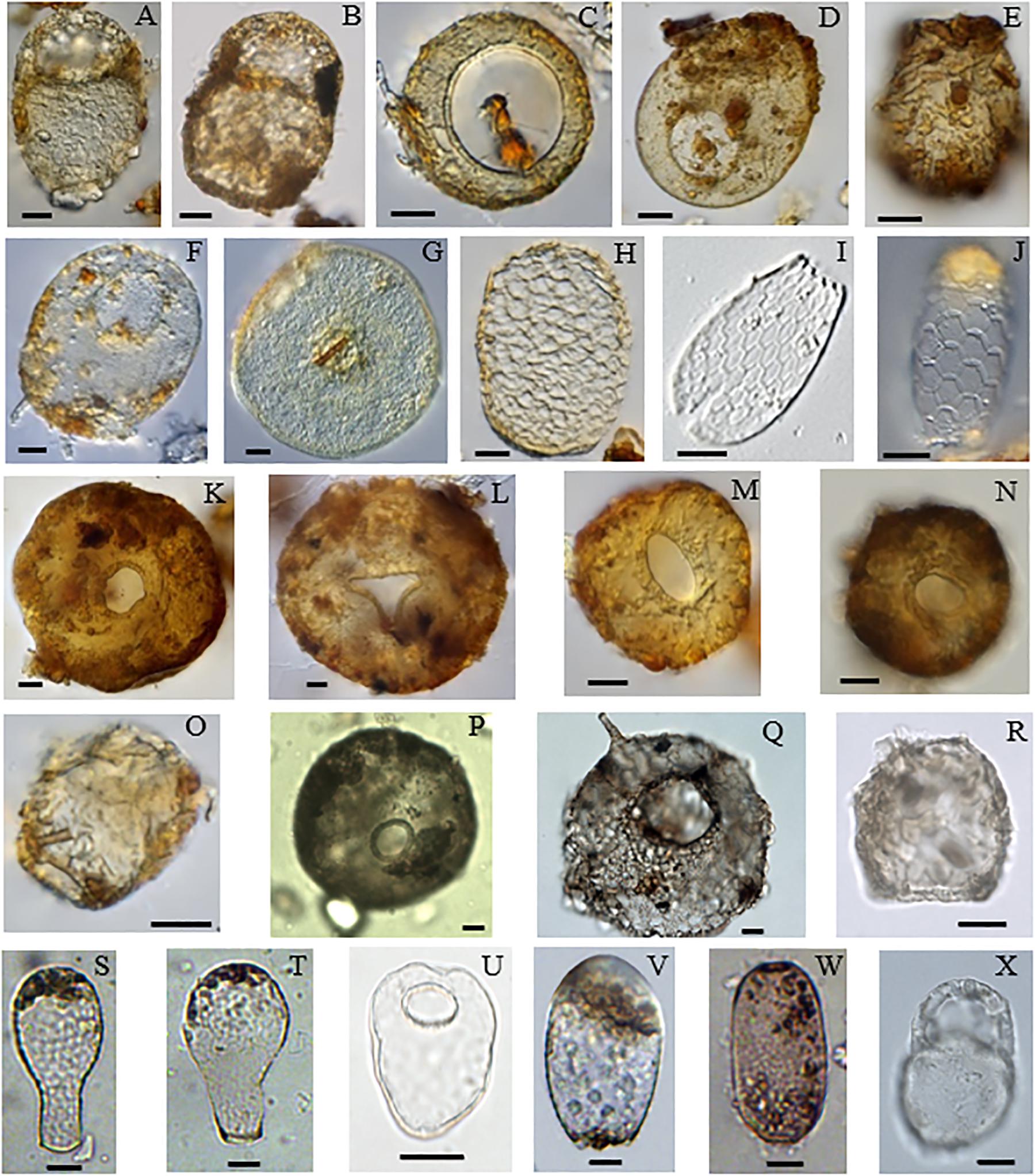
Figure 6. Some taxa found in the samples. (A) Centropyxis constricta, (B) Centropyxis aerophila morph 1, (C) cf. Cyclopyxis eurystoma v. parvula morph 2, (D) Centropyxis aculeata oblonga morph 2, (E) Difflugia penardi morph 1, (F) Centropyxis aculeata oblonga morph 1, (G) Arcella arenaria v. compressa, (H) Heleopera sylvatica, (I) Euglypha rotunda, (J) Tracheleuglypha dentata morph 2, (K) Trigonopyxis sp1 morph 3, (L) Trigonopyxis arcula v. major, (M) Cyclopyxis sp1 morph 1, (N) Cyclopyxis sp1 morph 2, (O) cf. Cyclopyxis eurystoma f. alta, (P) cf. Trigonopyxis microstoma morph 1, (Q) Centropyxis aculeata morph 2, (R) Difflugia angulostoma, (S) Padaungiella tubulata morph 1, (T) Padaungiella wailesi morph 1, (U) Trinema complanatum morph 2, (V) Jungia sundanensis cf. ovoidalis, (W) Archerella flavum, and (X) Centropyxis platistoma morph 2. For taxa details, see Supplementary Table 2. Scale bares = 10 μm. Image A-O by F. Siemensma, P-X by A.S. Esaulov.
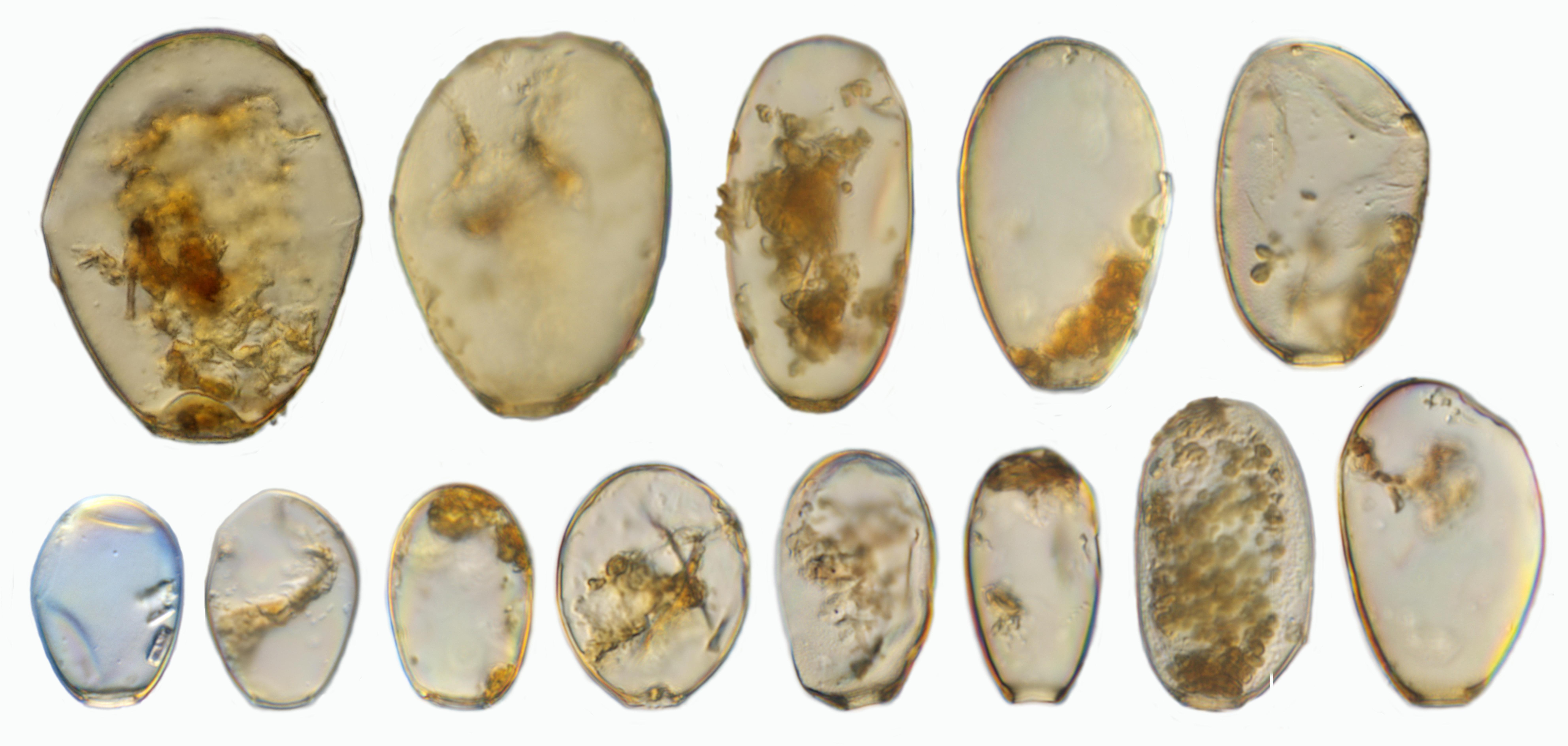
Figure 7. Examples of different Hyalopshenia subflava morphotypes with a shell length of 40 to 105 μm from one location (NS13). Image by F. Siemensma.
The most diverse genus was Centropyxis with about 28 morphotypes, however, it represented only 1.9% of the total abundance of testate amoebae. We did not find any species restricted to the Southern Hemisphere that is in contrast to the study of Hoogenraad and de Groot (1940), which reported Apodera vas, a typical Southern Hemisphere species, in the Kerinci mountains in Sumatra. However, we also found some endemic species such as C. minuta with an elliptic aperture (Bartos, 1963), Jungia sundanensis cf. ovoidalis, J. intermedia as well as cf. Trigonopyxis microstoma, which was also characterised by a pronounced shell variability (Figure 6). Surprisingly, we found only two shells of Archerella flavum and A. jollyi, which are very common in high latitude peatlands, but very rare in peatlands in New Zealand and absent in other peatlands of the Southern Hemisphere (Charman, 1997; Charman et al., 2000; van Bellen et al., 2014). Further, twelve taxa could not be determined to the morphospecies level, and twelve taxa were ambiguous, therefore they were signed as similar to the ones known (cf.), see Figure 6.
Relationships of Testate Amoeba Morphospecies and Traits With Environmental Variables
From all measured environmental variables, only WTD and pH explained a significant fraction of the variation in morphotype composition. For the development of transfer functions, WTD with its wide range (from 0 to 120 cm) seems to be more appropriate than pH with its restricted variation (from 2.5 to 3.8). Further, our study once again showed that the substrate water content is inferior to the WTD in explaining community composition of testate amoebae (Charman and Blundell, 2007; van Bellen et al., 2014). This can be explained by a greater stability of WTD in comparison to surface wetness. Supporting the suggestion of Swindles et al. (2018a), our results underline the sensitivity of testate amoebae to hydrological fluctuation in tropical ecosystems.
The wet localities at our study site were dominated by C. cf. aculeata oblonga morph 3, C. kahli, C. cf. aplanata microstoma morph 1, cf. T. microstoma and Spenoderia lenta morph 1. Similarly, C. aculeata have been suggested to indicate wet conditions in other regions (Swindles et al., 2018a, b). C. aculeata WTD optima, ranging between 20 and 30 cm, were similar to those from Continental Canada (Charman and Warner, 1992), West Russia (Bobrov et al., 1999) and Panama (Swindles et al., 2018a). Thus, for wet indicators such as C. aculeata, which are well known from the Northern Hemisphere, we confirm their value as an indicator of wet conditions in tropics. In addition, the WTD optimum of S. lenta at our study site (27.4 cm) was similar to that reported from a bog in Continental Canada [22.4 cm; Charman and Warner (1992)] and Patagonian peatlands in the Southern Hemisphere [ca. 32.0 cm; van Bellen et al. (2014)]. In contrast to the well-known and globally distributed C. aculeata, little is known about the ecology of rare species such as cf. T. microstoma (Figure 6P). T. microstoma has only been recorded from southern Patagonia (Argentina and Chile), Ecuador, Vietnam and New Zealand. The WTD optimum for this species has only been reported from south Patagonia (15 cm), which is similar to 20 cm optimum of smaller morphotype of cf. T. microstoma morph 1 (Hoogenraad and de Groot, 1948; Bartos, 1963; Zapata et al., 2008; Bobrov et al., 2010; Krashevska et al., 2012; van Bellen et al., 2014). We suggest considering cf. T. microstoma as an indicator for of wet conditions. Notably, for our individuals of cf. T. microstoma, the shell-to-aperture ratio was lower (4.6) compared to the originally described ones (8.2) by Hoogenraad and de Groot (1948), therefore this morphospecies may well be classified as a new species.
Dry locations in the studied peatland were dominated by a number of morphospecies of Trinema and Euglypha, which is in line with the results of the studies from North America and Europe (Charman et al., 2000). Notably, preferences for WTD depended on the size of the morphotypes, i.e., shells were larger in wetter locations. For example, T. arcula with large shells (130 μm) had a WTD optimum at 40.8 cm, whereas smaller morphotypes (95 μm) had their optimum at 63.9 cm. Similar patterns were observed by Schulz et al. (2018) in another tropical region, Ecuadorian Andes, showing that the shells of T. arcula were smaller in habitats characterised by low precipitation and low water availability. The WTD optima of the two smaller morphotypes of T. arcula (morph 1 and morph 2) were similar to the previously reported values (60 cm) in the Jura Mountains (Mitchell et al., 2001). Furthermore, the shell size of Trigonopyxis morphotypes with irregular aperture (similar to T. arcula major; Figure 4) decreases from 95 to 84 to 64 μm parallel to the increase in WTD optimum from 32.7 to 69.7 to 76.6 cm.
The WTD optima of the most abundant morphospecies H. subflava ranged from 27.5 to 74.1 cm. Small morphotypes, such as H. subflava morph 1 and morph 2, dominated in dry locations, whereas larger H. subflava morph 3, 5, 7, and 10 were abundant in wet locations. Previous studies suggested the WTD optimum of for H. subflava is 14.9 cm in peatlands of New Zealand (Charman, 1997) and 59.0 cm in bogs of the Jura Mountains in Switzerland (Mitchell et al., 2001). Thus, the indicator value of H. subflava needs to be reconsidered for tropical regions. Different optima of the morphotypes of H. subflava in the studied peatland indicate strong sensitivity of these taxa to WTD fluctuations. Therefore, we assume that the large variation in shell size of H. subflava, i.e., the co-existence of morphotypes differing in shell size in the studied peatland, is an indicator of an unstable hydrological regime. This is in line with the observation of Sullivan and Booth (2011) and Swindles et al. (2014) showing that some taxa, including H. subflava, are more abundant at more variable hydrological conditions.
For the second most abundant species complex, cf. C. eurystoma v. parvula morph 2 and morph 3, WTD optima varied from 53.2 to 62.2 cm and for cf. C. eurystoma v. parvula morph 1, the WTD optimum was at 75.9 cm. In comparison, the optima of cf. C. eurystoma v. parvula in ombrotrophic and minerotrophic peatlands of the southern taiga of West Siberia were estimated as 27.6 cm and 6.9 cm, in the peats with WTD range of 0 to 46 cm (Kurina and Li, 2019).
In our samples we found representatives of the genera Pyxidicula and Microchlamys as well as species Tracheleuglypha dentata, Centropyxis ecornis and Nebela collaris, which are known to preferentially inhabit rich fens (rich in calcium, with pH range from 6 to 8, but nutrient-poor), while in poor fens (nutrient poor, slightly acidic) and bogs they have not been recorded (see Marcisz et al., 2020, submitted). However, pH values in the studied peatland were very low (indicating ombrotrophic conditions), but C-to-N ratio of the substrate was low too, potentially indicating slightly minerotrophic conditions at least at some sampling points (Andersson et al., 2012). Such sites are typically characterised by the absence or low abundance of mixotrophic testate amoeba species (Jassey et al., 2014).
Again, we want to emphasise that some of the studied testate amoeba taxa have similar hydrological preferences across the globe, whereas others do not. Documentation of these differences, as well as the preferences of rare species with restricted geographical distribution, and the survey of species in underrepresented in literature tropical regions needs more attention. Further, species complexes, such as Trigonopyxis and Hyalosphenia, should be investigated in more detail including morphological and genetic traits potentially related to their hydrological preferences that may explain their wide range of hydrological tolerance.
Similar to morphotypes, only WTD and pH explained a significant fraction of the variation in the selected functional traits composition. Shell length, shell width and shell biovolume (which all were correlated) showed the strongest negative correlation with the WTD. In line with the results of our study, the predominance of smaller shells of testate amoebae at higher WTDs was shown in a field manipulation experiment (Koenig et al., 2018). Furthermore, testate amoebae with oviform/elongate shells dominated in dry biotopes, while sub-spherical testate amoebae (shell compression) and those with oval/circular aperture (aperture shape) were common in wet locations. This is similar to other studies, which showed that compressed shells and shells with small aperture preferentially occurred in dry habitats (Fournier et al., 2016; van Bellen et al., 2017; Koenig et al., 2018). By contrast, aperture position, presence of mineral particles on shell surface or mixotrophy did not correlate with WTD, with the latter being barely surprising as the abundance of mixotrophs was low (Lamentowicz et al., 2015; van Bellen et al., 2017).
Morphotype- and Trait-Based Transfer Functions
Both leave-one-out and bootstrap cross-validation provided relatively low model performance for the WTD transfer function based on morphotype data (RMSEP varied from 15 to 16 cm). By comparison, earlier studies on WTD transfer functions based on testate amoeba species showed RMSEP in the range of 2–8 cm (Payne and Mitchell, 2007; Booth et al., 2010; Swindles et al., 2018a). The wide range of WTD values in our study (0 to 120 cm) may explain this difference (van Bellen et al., 2014). The WTD gradient investigated in this study was 50% or even much wider than in most published training sets, e.g., over 60 cm in the study of Booth et al. (2010) and over 15 cm in the study of Payne and Mitchell (2007). Moreover, the RMSEP of our study is similar to that reported by van Bellen et al. (2014) (RMSEP 13 – 14 cm) in the ombrotrophic bogs in southern Patagonia which were characterised by a wide WTD range (0–104 cm). Notably, taking into account the WTD range, the relative errors in our study site (12.5–13.3%) showed values similar to the above-mentioned studies (11.5–14.5%), irrespective of the region. Thus, despite the high value of RMSEP in our study, the predictive power of WTD still remains at the same scale.
Weighted averaging resulted in the best model performance for the morphotype transfer function, whereas multiple regression gave the best model performance for functional traits. However, the trait-based transfer function provided lower model performance as compared to the morphotype-based one. The best RMSEP of 20.7 cm was obtained by including five traits in the model, i.e., aperture shape (oval/circular), aperture invagination (slightly invaginated), shell shape (oviform/elongate), shell compression (sub-spherical) and shell width. Among the traits related to the shell size, the shell width had a greater predictive power than others (Supplementary Table 6), even though shell width could probably be more casually related to the substrate wetness reflecting the width of the substrate pores. However, shell length may be a better indicator if used as intraspecific rather than interspecific trait, e.g., in Trigonopyxis and Hyalosphenia. Size stability in some species and variability in others may reflect the polyphyletic nature of testate amoebae, when shifts in shell size could have evolved differently in each lineage. Similarly, in oribatid mites it has been shown that phylogenetic signal of the body size and shape in some clades evolved independently several times but was conserved in others (Schaefer and Caruso, 2019).
Overall, RMSEP values of morphotype-based model (15–16 cm) and trait-based model (20.7 cm) are lower than the standard deviation of WTD in the studied peatland (33.4 cm), reflecting the predictive power of both transfer functions. The 4 cm difference in RMSEP between the morphotype- and the trait-based models is rather small considering the wide range of WTD (0–120 cm) in the studied peatland. The results of this study show that both morphotype-based and trait-based WTD transfer functions enable reliable reconstruction of palaeohydrological conditions in the study area, confirming the applicability of the trait-based approach. Thus, our weighted averaging transfer function will allow the first quantitative WTD reconstructions from a peat swamp in Central Sumatra. The trait-based reconstruction may be used to predict ecological preferences of unknown, rare or difficult for identification taxa. Moreover, considering that many morphospecies cannot be accurately determined taxonomically, the trait-based transfer function may provide additional realistic information avoiding inaccuracies due to identification errors and changing taxonomic affiliations.
Conclusion
The results of the present study contribute to the knowledge on testate amoebae in tropical ecosystems and indicate that for the development of transfer functions the ecological preferences of morphotypes within morphospecies also need to be considered and included in future studies. Moreover, there is an urgent need to fill the knowledge gap on geographical variations in the indicator value of cosmopolitan species to allow better palaeoecological reconstructions. Unfortunately, with few exceptions (Swindles et al., 2014, 2018a), transfer functions have only been established for European and North American peatlands until today. Overall, this study has presented the first testate amoebae transfer functions for reconstructing hydrological regime in the Southeast Asia and the third one based on functional traits. Our investigation also provided new insight into ecological preferences of a number of testate amoebae in tropical peatlands. Although hydrological preferences of some morphospecies were similar to those in high and middle latitudes, latitudinal differences in morphospecies and variations in environmental relationships of species require the development of region-specific transfer functions. Further, we intend to study subfossil testate amoebae from the Sungai Buluh peatlands and apply the developed transfer functions to reconstruct the palaeohydrological dynamics of peatlands in Sumatra with the scope to understand peat swamp ecosystem hydrological dynamics during the Holocene.
Data Availability Statement
All datasets presented in this study are included in the article/Supplementary Material.
Author Contributions
VK and SB conceived and designed the study. SB, VK, and AE performed the sampling and extractions. AE, VK, SB, and KH analysed the samples. VK and AT prepared and analysed the data and wrote the first draft of the manuscript. VK, YM, HB, and SB contributed reagents, material, and analysis tools. YM secured funding for microscopic and the data analyses and contributed to the data analysis methodology. AS, SS, SB, and HB provided field logistic support and acquired the funding. All authors contributed to writing and editing the manuscript and gave final approval for publication.
Funding
This study was financed by the Deutsche Forschungsgemeinschaft (DFG), project number 192626868–SFB 990 in the framework of the collaborative German – Indonesian research project CRC990. Microscopic and data analyses were supported by the Russian Science Foundation (grant 19-14-00102).
Conflict of Interest
The authors declare that the research was conducted in the absence of any commercial or financial relationships that could be construed as a potential conflict of interest.
Acknowledgments
We gratefully acknowledge the Ministry of Research and Technology of Indonesia (RISTEK) for the research permits, the Indonesian Institute of Sciences (LIPI) for collection permits, and the Ministry of Forestry (PHKA) for the export permit. We thank for logistic support the EFForTS coordination team and the Indonesian partner universities, Institut Pertanian Bogor (IPB) and University of Jambi (UNJA), the Ministry of Education in Jakarta (DIKTI) and LIPI. We thank the many colleagues and helpers for their support in the field and assistance in the laboratory. Special thanks to Handriyani, Erik Suwananda, Reza Mardhony, and Vera Alluioningruni for their assistance in the field.
Supplementary Material
The Supplementary Material for this article can be found online at: https://www.frontiersin.org/articles/10.3389/fevo.2020.00225/full#supplementary-material
References
Aldrian, E., and Susanto, D. R. (2003). Identification of three dominant rainfall regions within Indonesia and their relationship to sea surface temperature. Int. J. Climatol. 23, 1435–1452. doi: 10.1002/joc.950
Amesbury, M. J., Booth, R. K., Roland, T. P., Bunbury, J., Clifford, M. J., Charman, D. J., et al. (2018). Towards a Holarctic synthesis of peatland testate amoeba ecology: development of a new continental-scale palaeohydrological transfer function for North America and comparison to European data. Quat. Sci. Rev. 201, 483–500. doi: 10.1016/j.quascirev.2018.10.034
Amesbury, M. J., Swindles, G. T., Bobrov, A., Charman, D. J., Holden, J., Lamentowicz, M., et al. (2016). Development of a new pan-European testate amoeba transfer function for reconstructing peatland palaeohydrology. Quat. Sci. Rev. 152, 132–151. doi: 10.1016/j.quascirev.2016.09.024
Andersson, R. A., Meyers, P., Hornibrook, E., Kuhry, P., and Mörth, C.-M. (2012). Elemental and isotopic carbon and nitrogen records of organic matter accumulation in a Holocene permafrost peat sequence in the East European Russian Arctic. J. Quat. Sci. 27, 545–552. doi: 10.1002/jqs.2541
Biagioni, S., Krashevska, V., Achnopha, Y., Saad, A., Sabiham, S., and Behling, H. (2015). 8000 years of vegetation dynamics and environmental changes of a unique inland peat ecosystem of the Jambi Province in Central Sumatra, Indonesia. Palaeogeogr. Palaeoclimatol. Palaeoecol. 440, 813–829. doi: 10.1016/j.palaeo.2015.09.048
Birks, H. J. B., Line, J. M., Juggins, S., Stevenson, A. C., and Braak, C. J. F. T. (1990). Diatoms and pH reconstruction. Philos. Trans. R. Soc. B Biol. Sci. 327, 263–278. doi: 10.1098/rstb.1990.0062
Bobrov, A. A., Charman, D. J., and Warner, B. G. (1999). Ecology of testate amoebae (Protozoa: Rhizopoda) on peatlands in western Russia with special attention to niche separation in closely related taxa. Protist 150, 125–136. doi: 10.1016/S1434-4610(99)70016-7
Bobrov, A. A., Mazei, Y. A., and Tiunov, A. V. (2010). Testate amoebae of a monsoon tropical forest of South Vietnam. Acta Protozool. 49, 311–325.
Booth, R. K., Lamentowicz, M., and Charman, D. J. (2010). Preparation and analysis of testate amoebae in peatland palaeoenvironmental studies. Mires Peat 7, 1–7.
Charman, D. J. (1997). Modelling hydrological relationships of testate amoebae (Protozoa: Rhizopoda) on New Zealand peatlands. J. R. Soc. New Zeal. 27, 465–483. doi: 10.1080/03014223.1997.9517549
Charman, D. J. (2001). Biostratigraphic and palaeoenvironmental applications of testate amoebae. Quat. Sci. Rev. 20, 1753–1764. doi: 10.1016/S0277-3791(01)00036-1
Charman, D. J., and Blundell, A. (2007). A new European testate amoebae transfer function for palaeohydrological reconstruction on ombrotrophic peatlands. J. Quat. Sci. 22, 209–221. doi: 10.1002/jqs.1026
Charman, D. J., Hendon, D., and Woodland, W. A. (2000). The Identification of Testate Amoebae (Protozoa: Rhizopoda) in Peats: QRA Technical Guide No. 9. London: Quaternary Research Association, doi: 10.1016/j.quascirev.2004.02.012
Charman, D. J., and Warner, B. G. (1992). Relationship between testate amoebae (Protozoa: Rhizopoda) and microenvironmental parameters on a forested peatland in northeastern Ontario. Can. J. Zool. 70, 2474–2482. doi: 10.1139/z92-331
Clough, Y., Krishna, V. V., Corre, M. D., Darras, K., Denmead, L. H., Meijide, A., et al. (2016). Land-use choices follow profitability at the expense of ecological functions in Indonesian smallholder landscapes. Nat. Commun. 7:13137. doi: 10.1038/ncomms13137
Fournier, B., Coffey, E. E. D., van der Knaap, W. O., Fernández, L. D., Bobrov, A., and Mitchell, E. A. D. (2016). A legacy of human-induced ecosystem changes: spatial processes drive the taxonomic and functional diversities of testate amoebae in Sphagnum peatlands of the Galápagos. J. Biogeogr. 43, 533–543. doi: 10.1111/jbi.12655
Fournier, B., Lara, E., Jassey, V. E., and Mitchell, E. A. (2015). Functional traits as a new approach for interpreting testate amoeba palaeo-records in peatlands and assessing the causes and consequences of past changes in species composition. Holocene 25, 1375–1383. doi: 10.1177/0959683615585842
Gehrels, W. R. (2000). Using foraminiferal transfer functions to produce high-resolution sea-level records from salt-marsh deposits, Maine, USA. Holocene 10, 367–376. doi: 10.1191/095968300670746884
Gilbert, D., Amblard, C., Bourdier, G., and Francez, A. J. (1998). The microbial loop at the surface of a peatland: structure, function, and impact of nutrient input. Microb. Ecol. 35, 83–93. doi: 10.1007/s002489900062
Graham, L. L. B., Giesen, W., and Page, S. E. (2017). A common-sense approach to tropical peat swamp forest restoration in Southeast Asia. Restor. Ecol. 25, 312–321. doi: 10.1111/rec.12465
Gutierrez-Martinez, J.-M., Castillo-Martinez, A., Medina-Merodio, J.-A., Aguado-Delgado, J., and Martinez-Herraiz, J.-J. (2017). Smartphones as a light measurement tool: case of study. Appl. Sci. 7:616. doi: 10.3390/app7060616
Hapsari, K. A., Biagioni, S., Jennerjahn, T. C., Reimer, P. M., Saad, A., Achnopha, Y., et al. (2017). Environmental dynamics and carbon accumulation rate of a tropical peatland in Central Sumatra, Indonesia. Quat. Sci. Rev. 169, 173–187. doi: 10.1016/j.quascirev.2017.05.026
Hoogenraad, H. R., and de Groot, A. A. (1940). Moosbewohnende thekamoebe rhizopoden von java und sumatra. Treubia Deel 17, 209–259.
Hoogenraad, H. R., and de Groot, A. A. (1942). On fossil freshwater Rhizopoda from tropical moors in Sumatra and Borneo. Proc. K. Ned. Akad. Wet. C. 45, 734–741.
Hoogenraad, H. R., and de Groot, A. A. (1948). Thecamoebous Moss-rhizopods from New Zealand. Hydrobiologia 1, 28–44. doi: 10.1007/bf02519268
Jackson, S. T., and Charman, D. (2010). Editorial: peatlands - paleoenvironments and carbon dynamics. PAGES News 18, 3–4. doi: 10.22498/pages.18.1.3
Jassey, V. E. J., Lamentowicz, L., Robroek, B. J. M., Gabka, M., Rusińska, A., and Lamentowicz, M. (2014). Plant functional diversity drives niche-size-structure of dominant microbial consumers along a poor to extremely rich fen gradient. J. Ecol. 102, 1150–1162. doi: 10.1111/1365-2745.12288
Juggins, S. (2017). rioja: Analysis of Quaternary Science Data. R package Version (0.9-21). Available online at: http://cran.r-project.org/package=rioja.
Juggins, S., and Birks, H. J. B. (2012). “Quantitative environmental reconstructions from biological data,” in Tracking Environmental Change Using Lake Sediments. Volume 5: Data Handling and Numerical Techniques, eds H. J. B. Birks, A. F. Lotter, S. Juggins, and J. P. Smol (Dordrecht: Springer), 431–494. doi: 10.1007/978-94-007-2745-8_14
Karger, D. N., Conrad, O., Böhner, J., Kawohl, T., Kreft, H., Soria-Auza, R. W., et al. (2016). CHELSA Climatologies at High Resolution for the Earth’s Land Surface Areas (Version 1.1) World Data Cent. Clim. World Data Center for Climate (WDCC). Hamburg: DKRZ, doi: 10.1594/WDCC/CHELSA_v1_1
Klemm, J., Herzschuh, U., Pisaric, M. F. J., Telford, R. J., Heim, B., and Pestryakova, L. A. (2013). A pollen-climate transfer function from the tundra and taiga vegetation in Arctic Siberia and its applicability to a Holocene record. Palaeogeogr. Palaeoclimatol. Palaeoecol. 386, 702–713. doi: 10.1016/j.palaeo.2013.06.033
Kleyer, M., Dray, S., Bello, F., Lepš, J., Pakeman, R. J., Strauss, B., et al. (2012). Assessing species and community functional responses to environmental gradients: which multivariate methods? J. Veg. Sci. 23, 805–821. doi: 10.1111/j.1654-1103.2012.01402.x
Koenig, I., Mulot, M., and Mitchell, E. A. D. (2018). Taxonomic and functional traits responses of Sphagnum peatland testate amoebae to experimentally manipulated water table. Ecol. Indic. 85, 342–351. doi: 10.1016/j.ecolind.2017.10.017
Krashevska, V., Klarner, B., Widyastuti, R., Maraun, M., and Scheu, S. (2016). Changes in structure and functioning of protist (testate amoebae) communities due to conversion of lowland rainforest into rubber and oil palm plantations. PLoS One 11:e0160179. doi: 10.1371/journal.pone.0160179
Krashevska, V., Sandmann, D., Maraun, M., and Scheu, S. (2012). Consequences of exclusion of precipitation on microorganisms and microbial consumers in montane tropical rainforests. Oecologia 170, 1067–1076. doi: 10.1007/s00442-012-2360-6
Kurina, I. V., and Li, H. (2019). Why do testate amoeba optima related to water table depth vary? Microb. Ecol. 77, 37–55. doi: 10.1007/s00248-018-1202-4
Laliberté, E., Legendre, P., and Shipley, B. (2014). FD: Measuring Functional Diversity from Multiple Traits, and Other Tools for Functional Ecology. R Packag. Version, 1.0-12. Available online at: https://rdrr.io/cran/FD/
Lamentowicz, M., Gałka, M., Lamentowicz, Ł, Obremska, M., Kühl, N., Lücke, A., et al. (2015). Reconstructing climate change and ombrotrophic bog development during the last 4000 years in northern Poland using biotic proxies, stable isotopes and trait-based approach. Palaeogeogr. Palaeoclimatol. Palaeoecol. 418, 261–277. doi: 10.1016/j.palaeo.2014.11.015
Massaferro, J., and Larocque-Tobler, I. (2013). Using a newly developed chironomid transfer function for reconstructing mean annual air temperature at Lake Potrok Aike, Patagonia, Argentina. Ecol. Indic. 24, 201–210. doi: 10.1016/j.ecolind.2012.06.017
Mazei, Y., Chernyshov, V., Tsyganov, A. N., and Payne, R. J. (2015). Testing the effect of refrigerated storage on testate amoeba samples. Microb. Ecol. 70, 861–864. doi: 10.1007/s00248-015-0628-1
Mazei, Y. A., Blinokhvatova, Y. V., and Embulaeva, E. A. (2011). Specific features of the microspatial distribution of soil testate amoebae in the forests of the Middle Volga region. Arid Ecosyst. 1, 46–52. doi: 10.1134/S2079096111010069
Melati, D. N., Nengah Surati Jaya, I., Pérez-Cruzado, C., Zuhdi, M., Fehrmann, L., Magdon, P., et al. (2015). “Spatio-temporal analysis on land transformation in a forested tropical landscape in Jambi Province, Sumatra,” in Proceedings of the Conference Presentation of European Geosciences Union General Assembly 17 April 2015 (Vienna: EGU General Assembly).
Miettinen, J., Hooijer, A., Shi, C., Tollenaar, D., Vernimmen, R., Liew, S. C., et al. (2012). Extent of industrial plantations on Southeast Asian peatlands in 2010 with analysis of historical expansion and future projections. GCB Bioener. 4, 908–918. doi: 10.1111/j.1757-1707.2012.01172.x
Miettinen, J., Wang, J., Hooijer, A., and Liew, S. (2013). Peatland conversion and degradation processes in insular southeast Asia: a case study in Jambi, Indonesia. L. Degrad. Dev. 341, 334–341. doi: 10.1002/ldr.1130
Mitchell, E. A. D., Charman, D. J., and Warner, B. G. (2008a). Testate amoebae analysis in ecological and paleoecological studies of wetlands: past, present and future. Biodivers. Conserv. 17, 2115–2137. doi: 10.1007/s10531-007-9221-3
Mitchell, E. A. D., Payne, R., and Lamentowicz, M. (2008b). Potential implications of differential preservation of testate amoeba shells for paleoenvironmental reconstruction in peatlands. J. Paleolimnol. 40, 603–618. doi: 10.1007/s10933-007-9185-z
Mitchell, E. A. D., Lamentowicz, M., Payne, R. J., and Mazei, Y. (2014). Effect of taxonomic resolution on ecological and palaeoecological inference – a test using testate amoeba water table depth transfer functions. Quat. Sci. Rev. 91, 62–69. doi: 10.1016/j.quascirev.2014.03.006
Mitchell, E. A. D., van der Knaap, W. O., van Leeuwen, J. F. N., Buttler, A., Warner, B. G., and Gobat, J.-M. (2001). The palaeoecological history of the Praz-Rodet bog (Swiss Jura) based on pollen, plant macrofossils and testate amoebae (Protozoa). Holocene 11, 65–80. doi: 10.1191/095968301671777798
Nurjanah, S., Octavia, D., and Kusumadewi, F. (2013). “Identifikasi lokasi penanaman kembali ramin (Gonystilus bancanus Kurz) di hutan rawa gambut Sumatra dan Kalimantan,” in Pus. Penelit. dan Pengemb. Konserv. dan Rehabil. with Int. Trop. Timber Organ. (ITTO)-CITES Phase 2 (Indonesia).
Oksanen, J., Blanchet, F., Guillaume Friendly, M., Kindt, R., Legendre, P., McGlinn, D., et al. (2017). vegan: Community Ecology Package. R package version 2.4-5. Available online at: https://CRAN.R-project.org/package=vegan
Page, S. E., Rieley, J. O., and Banks, C. J. (2011). Global and regional importance of the tropical peatland carbon pool. Glob. Chang. Biol. 17, 798–818. doi: 10.1111/j.1365-2486.2010.02279.x
Payne, R. J., and Mitchell, E. A. D. (2007). Ecology of testate amoebae from mires in the Central Rhodope Mountains, Greece and development of a transfer function for palaeohydrological reconstruction. Protist 158, 159–171. doi: 10.1016/j.protis.2006.11.003
Posa, M. R. C., Wijedasa, L. S., and Corlett, R. T. (2011). Biodiversity and conservation of tropical peat swamp forests. Bioscience 61, 49–57. doi: 10.1525/bio.2011.61.1.10
R Core Team (2018). R: A Language and Environment for Statistical Computing. Vienna: R Foundation for Statistical Computing.
Saji, N. H., Goswami, B. N., Vinayachandran, P. N., and Yamagata, T. (1999). A dipole mode in the tropical Indian Ocean. Nature 401, 360–363. doi: 10.1038/43854
Schaefer, I., and Caruso, T. (2019). Oribatid mites show that soil food web complexity and close aboveground-belowground linkages emerged in the early Paleozoic. Commun. Biol. 2:387. doi: 10.1038/s42003-019-0628-7
Schulz, G., Maraun, M., Völcker, E., Scheu, S., and Krashevska, V. (2018). Evaluation of morphological characteristics to delineate taxa of the Genus Trigonopyxis (Amoebozoa, Arcellinida). Protist 169, 190–205. doi: 10.1016/j.protis.2018.02.005
Simpson, G. L. (2007). Analogue methods in palaeoecology: using the analogue package. J. Stat. Softw. 22, 1–29.
Sullivan, M. E., and Booth, R. K. (2011). The potential influence of short-term environmental variability on the composition of testate amoeba communities in sphagnum peatlands. Microb. Ecol. 62, 80–93. doi: 10.1007/s00248-011-9875-y
Swindles, G. T., Baird, A. J., Kilbride, E., Low, R., and Lopez, O. (2018a). Testing the relationship between testate amoeba community composition and environmental variables in a coastal tropical peatland. Ecol. Indic. 91, 636–644. doi: 10.1016/j.ecolind.2018.03.021
Swindles, G. T., Kelly, T. J., Roucoux, K. H., and Lawson, I. T. (2018b). Response of testate amoebae to a late Holocene ecosystem shift in an Amazonian peatland. Eur. J. Protistol. 64, 13–19. doi: 10.1016/j.ejop.2018.03.002
Swindles, G. T., Lamentowicz, M., Reczuga, M., and Galloway, J. M. (2016). Palaeoecology of testate amoebae in a tropical peatland. Eur. J. Protistol. 55, 181–189. doi: 10.1016/j.ejop.2015.10.002
Swindles, G. T., Reczuga, M., Lamentowicz, M., Raby, C. L., Turner, T. E., Charman, D. J., et al. (2014). Ecology of testate amoebae in an Amazonian peatland and development of a transfer function for palaeohydrological reconstruction. Microb. Ecol. 68, 284–298. doi: 10.1007/s00248-014-0378-5
Swindles, G. T., and Roe, H. M. (2007). Examining the dissolution characteristics of testate amoebae (Protozoa: Rhizopoda) in low pH conditions: implications for peatland palaeoclimate studies. Palaeogeogr. Palaeoclimatol. Palaeoecol. 252, 486–496. doi: 10.1016/j.palaeo.2007.05.004
Tata, H. L., van Noordwijk, M., Jasnari, and Widayati, A. (2016). Domestication of Dyera polyphylla (Miq.) Steenis in peatland agroforestry systems in Jambi, Indonesia. Agrofor. Syst. 90, 617–630. doi: 10.1007/s10457-015-9837-3
van Bellen, S., Mauquoy, D., Payne, R. J., Roland, T. P., Daley, T. J., Hughes, P. D. M., et al. (2014). Testate amoebae as a proxy for reconstructing Holocene water table dynamics in southern Patagonian peat bogs. J. Quat. Sci. 29, 463–474. doi: 10.1002/jqs.2719
van Bellen, S., Mauquoy, D., Payne, R. J., Roland, T. P., Hughes, P. D. M., Daley, T. J., et al. (2017). An alternative approach to transfer functions? Testing the performance of a functional trait-based model for testate amoebae. Palaeogeogr. Palaeoclimatol. Palaeoecol. 468, 173–183. doi: 10.1016/j.palaeo.2016.12.005
Wilmshurst, J. M., Wiser, S. K., and Charman, D. J. (2003). Reconstructing Holocene water tables in New Zealand using testate amoebae: differential preservation of tests and implications for the use of transfer functions. Holocene 13, 61–72. doi: 10.1191/0959683603hl595rp
Keywords: water table depth, protists, shell size, training set, Hylaosphenia, indicator, peat-swamp forest, hydrology
Citation: Krashevska V, Tsyganov AN, Esaulov AS, Mazei YA, Hapsari KA, Saad A, Sabiham S, Behling H and Biagioni S (2020) Testate Amoeba Species- and Trait-Based Transfer Functions for Reconstruction of Hydrological Regime in Tropical Peatland of Central Sumatra, Indonesia. Front. Ecol. Evol. 8:225. doi: 10.3389/fevo.2020.00225
Received: 14 April 2020; Accepted: 18 June 2020;
Published: 14 July 2020.
Edited by:
Vincent Jassey, UMR 5245 Laboratoire Ecologie Fonctionnelle et Environnement (ECOLAB), FranceReviewed by:
Bertrand Fournier, University of Potsdam, Institute of Environmental Science and Geography, Potsdam, GermanySimon Van Bellen, Université de Montréal, Canada
Copyright © 2020 Krashevska, Tsyganov, Esaulov, Mazei, Hapsari, Saad, Sabiham, Behling and Biagioni. This is an open-access article distributed under the terms of the Creative Commons Attribution License (CC BY). The use, distribution or reproduction in other forums is permitted, provided the original author(s) and the copyright owner(s) are credited and that the original publication in this journal is cited, in accordance with accepted academic practice. No use, distribution or reproduction is permitted which does not comply with these terms.
*Correspondence: Valentyna Krashevska, vkrashe@gwdg.de